Against Utopia Issue #6: The Epistemology of Depression Part 6 - What Does a Realistic Theory of Depression Look Like?
Hello, and welcome to Against Utopia, a newsletter about authoritarian rationalism in science, technology, politics, and medicine, and anti-authoritarian, decentralized, bottom-up alternatives. This is Issue Six, published August 2nd, 2019.
If you were forwarded this newsletter and want to subscribe, sign up here.
Enjoying the newsletter and want to support my work? Support me on Patreon.
Firstly, thanks for reading this far. The newsletter has a small audience of just over a couple hundred people, and almost all of you continue to read it and send me feedback and questions. Thanks for supporting my work!
A Quick Recap of Where What We’ve Covered
In Issue #1, we looked at how complex phenomena like depression must be simplified and objectified by authorities so that they can be assigned metrics and controlled. We saw how these phenomena are represented by simplistic, reductive features under the guise of utopian simplification and rationalism, and we quickly looked at how we know and see medical facts.
In Issue #2, we looked at how utopian simplification of the medical evidence for depression in humans isn’t reproduced well in whole organism animal studies, leaving a trail of messy, inconvenient facts that don’t add up with the low serotonin theory of depression.
In Issue #3, we unpacked how the apparatus of medical science perceives and develops facts about the body, via classification and taxonomies on one end, and empiricism and clinical experience on the other. We took a deep dive into the epistemic apparatus of doctors from the 18th century onward, and saw how these sometimes competing movements in nosology shaped the medical lens through which your doctor views your problems today—divorced from your experience, tallied up by statistics.
In Issue #4, we took a look at the principal drivers of knowledge production in medicine: the clinical trial, the surrogate endpoint, the risk factor, and the disembodiment of physiology by what I called the statistical-inferential medical gaze. I showed how the map of the body created by epidemiological risk factors does not significantly overlap with the territory of actual human physiology, and how as a result, we don’t really seem to cure anything anymore. We’ve become very good at endlessly identifying diseases and prescribing medication, but we’re no better off than we were decades ago when it comes to chronic illnesses.
In Issue #5, we learned how statistics, the pharma model of knowledge production (clinical trials), and a handful of misinterpretations early in the history of biogenic amine studies shaped a faulty medical perception of what serotonin does in the body. The combination of these misperceptions created an, at-best, broken clock theory of depression as caused by low serotonin. We then saw how medical authorities, in an effort to be more “objective”, used scientism to develop the DSM while simultaneously working to remove patient context and experience as a meaningful input to the pathological state of mental suffering, because it is too messy, subjective, non-systematic. This expanded the addressable market for who could be labeled as depressed and prescribed a drug, massively growing SSRI prescriptions and the pharmaceutical industry.
Thus far, my story is that the low serotonin theory of depression is an opportunistic theory - it’s supported more by poorly replicated randomized controlled trials, misinterpretations of these trials, and a human bureaucratic need to objectify, than objective knowledge in and of itself. This story makes more sense in light of the social, cultural, historical, epistemic, and ontological factors that produced the current system of medicalized depression, and the lack of evidence for the low serotonin theory that drives it. Let’s now apply this lens we’ve used to deconstruct the shoddiness of the low serotonin theory of depression, and see what a fruitful theory that better explains observations about serotonin’s role in depression actually looks like: the high serotonin theory of depression.
To be clear, there is no such thing as a “correct” theory of anything. All theories are idealizations that simplify or abstract away from reality to create a conceptual framework that is then used to make progress on a particular problem. The goal here has been to show that the current conceptual framework used to explain depression neglects oodles of evidence in mitochondrial biology and serotonin physiology. This framework is not an accident. It helps those who hold the reins of bureaucracy exercise regulatory legibility, medical tradecraft, administration, and institutional power to make tens of billions of dollars a year and exercise illegitimate authority over our biology. Anyway, let’s get into the meat of it.
The Low Serotonin Theory of Depression is Bunk
We’ve already covered the evidence against the low serotonin theory of depression, but here’s a quick reminder:
In black and brown bears, serotonin transmission is elevated seasonally in winter as resources dwindle. This elevation in serotonin transmission induces torpor, insulin resistance, fat storage, and eventually, hibernation, which fit many depressive phenotypes in humans. If serotonin is the happy molecule responsible for raising affect and mood and has us ready to tackle the day, we would expect the opposite of torpor if its transmission was increased.
In the red-cheeked ground squirrel, the enzyme system responsible for producing serotonin from tryptophan, tryptophan hydroxylase (TPH), becomes more active as environmental resources become scarce in the winter. The system produces more serotonin, raising serotonin serum levels as much as 50% in a matter of a couple days, dropping the body temperature, slowing the animal down and producing torpor, and finally, initiating hibernation. Again, if serotonin transmission is elevated, the low serotonin theory predicts that animals should become boisterous and gregarious, not torporific.
In the family of grasshoppers who can metamorphosize into locusts, variation in resource variability coupled with cramped quarters induces an increase in serotonin transmission via histamine deployment from scratching of hindlegs. This increase in serotonin transmission further induces metamorphosis, and slows the insects’ metabolism down, as a direct response to the perceived lack of resources. If serotonin were an “upper”, we’d expect gregariousness as the transmission of serotonin started, not after metamorphosis.
So, the low serotonin theory of depression is poorly supported once we include the studies in whole organisms. It appears that in all non-human animals, increasing serotonin transmission causes depression-like symptoms (torpor), instead of the other way around. More than anything else, it seems that in the animals studied, serotonin transmission is used to modulate energy production in response to stressful environmental conditions, real (experienced) or perceived. Let’s set this idea aside for the moment and come back to it in a little later on.
Human Studies Add More Holes to the Low Serotonin Theory
The story of serotonin as the causative agent in depression is only further complicated when we include human studies.
Some drugs that block serotonin reuptake at the post-synaptic neuron, such as cocaine and amphetamines, do not alleviate depression symptoms. We would expect drugs that block serotonin reuptake to robustly replicate the effects of e.g. fluoxetine, which works through the same purported mechanisms, but alas, they don’t, which means the picture is more complicated than high serotonin good, low serotonin bad.
In Issue #5, we covered the curious case of reserpine, an alkaloid isolated in 1955, believed to deplete biogenic amines such as serotonin thereby causing depressive symptoms. A placebo-controlled, randomized, parallel group study of reserpine in depressed people showed that reserpine actually alleviated depression. The physiological mechanism remained unchanged; it is still believed that reserpine depletes serotonin in the extracellular space, reducing serotonin transmission, yet the depressive symptoms abate nonetheless, suggesting that high serotonin is more likely to cause depression.
Though this is rather inconvenient (or is it? 🤔) for the pharmaceutical companies and the medical trade, SSRI drugs do not produce an antidepressant effect through manipulating serotonin levels at the synapse. Whether their pro-serotonin effects are actually the cause of depression is conveniently ignored because it takes an inordinately large amount of new evidence and a new explanatory paradigm typically to displace a very popular hypothesis. We don’t really have that yet in a strict, straightforward, randomized controlled trial because of many reasons, the top reason probably being no one has an incentive to fund a study that potentially kills a billion dollar drug for an entire industry.
Speaking of not manipulating serotonin levels at the synapse, selective serotonin reuptake inhibitors (SSRIs) act to increase serotonin transmission within minutes to hours somehow - but to the humans who take them, they do not seem to have any kind of reported, measurable effect until several weeks of continuous treatment. What is to explain this, the so-called therapeutic delay?
If increasing serotonin in the extracellular space alleviates depression by making serotonin more available, then why is it taking weeks to months for a beneficial effect to register in mental health measurement instruments like the Hamilton Depression Scale? The therapeutic delay remained largely unexplained, until recently. Taking a closer look at this only makes the holes in the low serotonin theory larger.
All successful antidepressants (and especially fluoxetine/Prozac) have two properties in common.
First, SSRIs increase synthesis of an endogenous steroid called allopregnanolone in the brain. This is such a well established fact that Prozac is the standard in animal trials that aim to study the effects of increased allopregnanolone synthesis.
Allopregnanolone has a proven, potent, rapid and long lasting antidepressant effect based on evidence accumulated over at least 50 years. The FDA just approved Brexanolone (a synthetic version of allopregnanolone) in March 2019 as a fast-acting, long-lasting antidepressant in humans for the treatment of postpartum depression. A single dose of this steroid produced antidepressant effects within 24 hours and those effects lasted for at least 30 days. Steroids have potent effects on cell signaling, and many of them also profoundly enhance mitochondrial energy production (more later on this).
Allopregnanolone (among other steroids) is also a selective serotonin antagonist at the 5-HT3 receptor. Antagonists on 5-HT3 like Ondansetron, an old drug used to treat motion sickness, vomiting, and gastroenteritis, have a solid amount of evidence behind them as potent antidepressants, especially in low doses (1mg-3mg daily for humans).
Second, all successful SSRI drugs are antagonists on the serotonin 5-HT2c receptor. This means that they actually bind the target of serotonin and prevent its binding to a degree (not 100%). Right away, we see a partial contradiction of what the official mechanism of action of SSRI drugs really is. Be that as it may, the 5-HT2c receptor is the master controller of the synthesis and release of adrenocorticotropic hormone (ACTH) in the pituitary gland. ACTH is made in response to stress in almost all organisms. The 5-HT2c receptor is a more potent inducer of ACTH than even corticotropin-releasing hormone (CRH) - the primary driver of ACTH release. These hormones all lead to very high levels of cortisol.
Now, why is this important?
High cortisol is perhaps the most widely accepted causative factor in depression both in the ritualistic trade organization-based circles we have been critiquing (doctors), and the metabolic-energetic theory of disease that we’ve been hinting at in the course of this series. Basically, by saying that serotonin is a modulator of energy production under stress, we are hinting at a metabolic origin of disease for anything it is linked to. Digging in a little further on high cortisol, the vast majority of depression patients have been shown to be non-responders to the "dexamethasone suppression test", a test used to show cortisol response under conditions of stress induced by injection of dexamethasone.
In other words, they have high cortisol and show signs of "cortisol resistance" because their cortisol does not drop in response to exogenously delivered cortisol. By giving them a potent antagonist on 5-HT2c like Prozac, you can watch their ACTH levels drop and then subsequently, their cortisol levels drop. Lowering cortisol has proven antidepressant effects in every single human trial to date.
(Sidenote: The abortion pill RU486 was actually developed as a glucocorticoid antagonist to treat Cushing’s syndrome and is used off-label to treat depression in many countries. However, the downregulation of cortisol synthesis and the subsequent increase in glucocorticoid receptor sensitivity by giving either an 5-HT2C antagonist like Prozac/fluoxetine or RU486 is known to take on the order of 3-4 weeks. This is further evidence that the therapeutic delay is not due to SSRI effects, but is instead due to effects from increasing the steroid allopregnanolone)
In addition, if you were instead to administer a 5-HT3 antagonist like ondansetron or allopregnanolone (which Prozac/fluoxetine increases), you’d produce an antidepressant effect after 4-6 weeks. On average, by giving a combined cortisol antagonist (through 5-HT2C) and 5-HT3 antagonist like Prozac/fluoxetine the expected antidepressant effect is not expected to kick in for at least 4-5 weeks.
And that is precisely how long the therapeutic delay for SSRI drugs is.
So to reinforce the point I’ve been trying to make all along - it’s not that SSRI drugs don’t work, it’s that they work in ways that are profoundly different from what the pharma-industrial complex claims, and the truth of the claim that SSRIs increase serotonin levels in order to alleviate depression reflects the need to drive the engine of prescriptions and revenue more so than any physiological truths about serotonin biology. There are much cheaper and simpler ways to alleviate depression with 5-HT3 antagonists, 5-HT2c antagonists, etc, but we’ll cover that in Issue #7.
…
But wait, there’s more! There may be additional mechanisms through which successful SSRI drugs work that are not under consideration yet because they are too controversial. For example, some of the older tricyclic antidepressants which do have SSRI properties in higher doses (e.g. amitryptilline, nortryptilline) are actually serotonin receptor antagonists when used in lower doses, and importantly, are also antagonists at the endotoxin receptor TLR4, which influence systemic inflammation.
Chronic inflammation has an extensive track record as a plausible causative factor in depression, which is why anti-inflammatory drugs like aspirin and ibuprofen have also successfully reduced depressive symptoms in small scale human trials for depression (more on this below). Given the tight positive feedback mechanism between TLR4 and serotonin synthesis and release in the gut (where more than 90% of all serotonin is produced), it is well-known that TLR4 antagonists will lower serotonin synthesis and release in the gut. This is yet another piece of direct evidence that opposing serotonin, by blocking its effect at the receptor level, or decreasing its synthesis and release has potent antidepressant effects.
Serotonin and dopamine, another biogenic amine, have a largely inverse relationship and feedback cycle. For example, dopamine antagonists are known to increase serotonin release, while dopamine agonists are known to lower serotonin release. Studies have found that these effects are due to dopamine and dopamine receptor agonists inhibiting tryptophan hydroxylase, one of the enzymes involved in the cascade that forms serotonin, directly. Most, if not all, dopamine antagonists / serotonin promoters cause depression as a known side effect. Conversely, every known dopamine agonist, also an inhibitor of serotonin synthesis, has potent antidepressant effects. This is one of the reasons patients tend to "abuse" dopamine agonists - not only do they improve sexual function but they have powerful mood lifting effects as well.
In yet another ancillary fashion, upstream of the direct action of serotonin is tryptophan, the amino acid precursor typically acquired via the diet (some people supplement 5-HTP and tryptophan directly in an effort to mitigate depressive effects - this is almost always a bad idea). If serotonin’s action is responsible for alleviating depression, then depleting tryptophan in the diet might be a viable strategy for inducing depression. Thus far, the attempt to induce depression by reducing tryptophan has failed in non-depressed participants in all attempts.
Another indirect pathway of increasing serotonin, via the serotonin transporter, SERT, offers further confounding evidence. Genetic downregulation of SERT, which increases the amount of serotonin left in the extracellular space, is associated with an increase in depressive symptoms, not a decrease.
Hopefully I’ve become a bore at this point: if the low serotonin theory of depression is correct, we should expect people with genetic downregulation of SERT to have increased resilience to depression, yet we observe the opposite.
What picture can we actually paint from the seemingly disparate facts gathered here? Doctors believe, and have led us to believe, that low serotonin causes depression. Almost every single intervention in animals and in humans disagrees with this position. So what phenomena actually begin to explain the biochemical observations, whole organism studies, human studies, and the experience of depression?
The High Serotonin Theory of Depression
In animal studies, when increased serotonin transmission is observed, we reliably observe the exact opposite intended effect of the low serotonin hypothesis. Animals slow down, become torporific, and in some cases, hibernate to build up their energy stores and make it through winter, under the direct influence of more serotonin at the synapse. The serotonergic system prepares the animal for real and perceived changes in resource status in the environment.
In other words, serotonin’s activity is tied to how much energy is available or is expected to be available in the animal’s environment, and this availability or perceived availability is integrated to regulate the energetic state of the animal, which includes how they behave, how much fat they store, whether they turn sugar into CO2 or lactic acid, down to the insulin sensitivity of their individual cells. Serotonin (and the serotonergic system in general) regulates energy production, particularly in response to energy availability in the face of environmental stress.
Second, in human studies, it seems that increasing serotonin transmission in the cell and increasing the availability of serotonin in the extracellular space acutely exacerbates depressive symptoms instead of abating them as expected.
The current hypothesis, the low serotonin theory of depression, is that the abatement of depression symptoms is achieved by, among other things, the production of allopregnanolone, which is an agonist of many serotonin receptors, while also rapidly producing anti-depressive effects (within 24 hours). Most SSRIs, like fluoxetine, take a few weeks to buildup and influence the production of allopregnanolone, which explains the therapeutic delay. SSRIs seem to increase serotonin and allopregnanolone, and you can conclude that most of their positive effects are probably not tied to increased serotonin at all, given what we know about how increased serotonin exacerbates depression symptoms in other animal models of depression and in humans, while increasing allopregnanolone rapidly extinguishes them (within 24 hours - how’s that for a delay!).
Furthermore, the delay refers to the fact that every single SSRI in existence will acutely exacerbate symptoms first, typically for a period of 4-8 weeks, before patients report an improvement in their mood on the Hamilton Depression Scale. The therapeutic delay is an inconvenient confounder of the low serotonin theory of depression, one that the pharma companies and the medical trade conveniently ignore or don’t actually understand, because they can still prescribe despite its existence. For the low serotonin theory to hold, you’d expect immediate improvement if biogenic amines like serotonin were alleviated depression, but it’s more likely that the complex behavior of inhibiting serotonin reuptake at the synapse has cascading effects that work for some phenotypes of depression, and not for others.
So we can conclude that, because SSRIs do not immediately effect depressive states, there is likely something else causing depressive symptoms. It’s not simply that low serotonin causes depression, because of the action of SSRIs. However, the picture gets a little clearer when we look at high serotonin states in experimental models and in humans.
First, in neonatal (infant) models of SSRI exposure in rats, there is a peculiar response that suggests that high serotonin is really at play in depression. Adult rats exposed to SSRIs as infants demonstrate increased serotonin transmission in the hypothalamus coupled with a depressive behavioral profile (they’re more sluggish, more inflamed, are less responsive to hedonic stimuli). As stated previously, we expect increased serotonin transmission to be associated with lower depression, and more gregariousness. Not so.
Continuing along this genetic approach, the Flinders Sensitive line of mice has been bred to exhibit many depressive symptoms. In this mice, serotonin and the ratio of 5-HIAA to 5-HTP, a measure of the metabolism of serotonin, is elevated in multiple regions, including the hypothalamus, the prefrontal cortex, and the hippocampus.
Other genetic models of depression include the SERT knockout and 5-HT1a receptor knockout rodents. These rodents have been bred without the ability to produce the SERT protein, which transports serotonin from the synaptic cleft to the presynaptic neuron. What this means in straightforward terms is that it reduces the serotonin concentration outside of the cell. When you knock out this gene, you produce a high serotonin state, and, surprise surprise, the rats become depressed.
I hope I’ve become a bore at this point. In many rather straightforward ways, the low serotonin theory of depression upon which many SSRI drugs are built, fails to explain the embodied physical behavior of the serotonergic system.
In the previous two sections we’ve seen how, amongst various animals including humans, serotonin taken in a behavioral perspective seems to help animals cope with stressful situations, whether they are real or perceived. For this, I’ll remind you what we reviewed with hibernation in bears, gregarization in locusts, and hibernation in ground squirrels.
Moving on down in scale, from the perspective of organ systems within these organisms, serotonin influences their energy expenditure, causing them to produce lactate from sugar via glycolysis, which causes them to literally slow down, produce less ATP, and begin to get fat and hibernate (in some cases). This causes scores of systemic changes in insulin sensitivity, glucose usage, and inflammation.
At the level of cells, if serotonin is influencing energy production, that means it is influencing mitochondria, the “powerhouses” of the cell which turn sugar into energy and power cellular energy metabolism. It is here that the picture gets even more interesting. Serotonin evolved in animals at least 200 million years ago, based on phylogenetic studies. In the last 20 years, we’ve become to piece together some of the evidence showing that serotonin’s original role in these systems was to exert a profound regulatory effect on energy production, making sure that the animal can preserve resources to respond to stressful situations and adapt to the environmental demands without dying. The interesting thing is that stressful is a relative, and contextual term. It will vary depending on the animal, the environment, the situation, and history (genetics to some extent).
And what we’ve been able to discover in the last 20 years or so has shown us that, in addition to depression’s metabolic and energetic dimensions, all affective disorders exhibit features of disordered or dysregulated energy metabolism. Everything from schizotypal disorders, anxiety, chronic fatigue syndrome, and more has been shown to be accompanied by mitochondrial inflammation, and energy dysregulation.
In order to bridge this knowledge gap between the behavioral and animal model observations we’ve talked about, and the sometimes-genetic, high serotonergic states that seem to be linked to depressive phenotypes, we should take a closer look at the behavioral typologies of human depression, and the curious way that deficits in energy metabolism always seem to accompany them.
Mitochondria, Serotonin, Energy Regulation, Stress, and Rumination
So, I’ve mentioned mitochondrial energy dysfunction and energy metabolism a few times to date. We haven’t really delved into it, other than acknowledging that failure to meet energetic demands (aka stress) seems to result in increased serotonin transmission, which can be accompanied by behavioral adaptations depending on the species of organism (e.g. gregarization in grasshoppers, hibernation in bears, depressive rumination in humans, etc).
If an organism’s energetic status is altered in response to the environment, this implies that the organism’s mitochondrial energy production is also altered. We’ve observed that serotonin alters energy metabolism in bears, grasshoppers, squirrels, and in humans, so we should be able to find evidence of mitochondrial energy production genetic mutations, alterations in chemical energy and products produced, or both. It turns out that’s exactly the case when we look a bit more closely at what is happening in mitochondria in affective spectrum disorders such as depression.
First, mitochondrial disorders themselves have common comorbidities with depression and affective spectrum disorders. Psychological stressors have frequently been observed in the triggering of depressive episodes in human patients and mice mitochondrial disorder models. Illness, fasting, over-exercise, and sexual exhaustion also have been shown to increase clinical symptamology of depression and other affective disorders. These contexts are always accompanied by higher brain energy demands for which the patient or animal model is not able to meet with adequate ATP production.
Abnormal central nervous system energy production is a major feature of all mitochondrial disorders. In one study of 36 patients with mitochondrial disorders, lifetime diagnoses of 54% for major depression, 17% for bipolar disorder, and 11% for panic disorder were reported. Two additional studies focusing on the genetic maternal inheritance of mitochondrial disorders demonstrated that there is a several-fold increased probability of developing depression in specific mitochondrial DNA (mtDNA) variants.
Several additional studies add more details to this view of mitochondrial dysfunction in depression. Postmortem brain studies of patients with diagnosed major depression disorder showed major deletions in 5kb mtDNA fragments. Key areas of the frontal, prefrontal, and tertiary visual cortices were affected by these deletions, as was one of the core areas of serotonin transmission in the brain, the dorsal raphe nucleus.
In mitochondria, there is a key functional area called the electron transport chain, which, roughly, shuttles electrons from sugar to oxygen to form carbon dioxide and ATP, the primary energy currency of the cell. In depressed people, there are marked decreases in the respiratory chain enzyme ratios and ATP production rates. Every depressed person in this study demonstrated low ATP production rates in biopsied muscle. Basically, this strongly suggests that depression is a systemic issue with features of impaired energy metabolism globally in a person, not just in the brain. The diagnosis of depression in a large majority to near totality of people with mitochondrial disorders further strengthens the connection: depressed people have demonstrably lower ATP production, and people with diagnosed diseases causing lower ATP production are more likely to be depressed.
So which way does the causality flow?
One way to answer that question is to ask what happens to anyone with a mitochondrial disorder when they are given a medication or supplement meant to support energy metabolism.
Coenzyme Q10 is an energy cofactor needed in various parts of the electron transport chain and respiratory complex in mitochondria. Coenzyme Q10 has been shown to be 50% lower in patients with treatment-resistant major depressive disorder.
A couple things happen when there isn’t enough CoQ10 to support oxidative metabolism. First, a lot of reactive oxygen species (ROS) are produced, sometimes orders of magnitude more compared to normatively healthy, non-depressed people. Second, this production of ROS also causes an increase in various inflammatory markers, including cytokines which are elevated in many depressive phenotypes (support for the role of pro-inflammatory cytokines in depression is the observation that they can induce depression in up to 70% of patients treated with such agents.
Supplementing with CoQ10 seems to reduce lactate and markers of oxidative stress. We won’t go too deeply into metabolism - entire books have been written about it. But suffice it to say for our purposes, reducing lactate can be thought of as “good” in a sense because lactate is produced by tissues under stress. As we learned in the section on bears in Issue #2, lactate is one of the precursors to insulin insensitivity, fat storage, and hibernation. It’s also elevated in diabetes, cancer, and heart disease, but I digress.
L-carnitine, an amino acid crucial to using fatty acids for energy production, has been shown to have a strong antidepressant effect, as strong as effects demonstrated by SSRIs.
Riboflavin demonstrates potent anti-cytokine behavior. Cytokines are upregulated in most depressive phenotypes. In addition, riboflavin seems to be broadly supportive for reducing inflammation associated with affective disorders resulting from migraine.
The “mitochondrial cocktail” has been trialed with patients suffering from inflammatory and affective disorders. In practice, it has been shown to reduce lactate production and lower cytokines and other markers of stress in all patients, while occasionally improving depressive symptoms. At this point, I’ll just quote Dr. Ann Gardner, who co-wrote and led the committee that approved the Diagnostic and Statistical Manual of Mental Disorders (DSM-IV). Think of her as largely setting the standard for how all psychiatrists treat mental and affective disorders.
“According to the experiences of both authors, we believe that the cocktail often demonstrates effects with the somatic symptoms in depression and possibly in depression itself. Efficacy usually does not occur for several months before there is an obvious improvement. Gold and Cohen suggest that “providing [mitochondrial cocktail] as part of an individual trial in which the patient serves as their own control seems to be a reasonable approach” (Gold and Cohen, 2001).”
Gardner, Gold, and Cohen believe the mitochondrial cocktail could be fruitful for depression and other affective disorders based on the evidence that the constituents of the cocktail help mitochondria produce carbon dioxide from sugar and oxygen, otherwise known as mitochondrial oxidative phosphorylation.
Thus far, we’ve primarily investigated the link between depression and serotonin, so what does all this mitochondria stuff have to do with that?
Serotonin is involved in metabolic energy switching, and rapid, dynamic adjustments in energy production depending on the organ, tissue, and system it is acting on. In particular, it seems to have a marked effect on the switching between oxidative phosphorylation and glycolysis.
Serotonin outside of the brain (95% of the pool in the body) regulates physiological function broadly and widely, in almost all types of cells. In the human pancreas, serotonin regulates beta cell function, the cells responsible for regulating sugar and fat homeostasis in the body. Depending on the context, serotonin appears to cause insulin insensitivity, a condition required for the development of type 2 diabetes.
Serotonin produced in the intestine acts on the liver to promote the production of sugar from other substrates (gluconeogenesis), a process that is triggered by environmental stress, and a failure to produce energy to meet demand (sound familiar?).
On fat cells, serotonin promotes lipolysis and free fatty acid release. This is a core feature of all mammalian responses to stress, as far back as the discovery of the general adaptation syndrome by Hans Selye. In an interesting twist, in brown fat adipocytes, a particular form of fat that is concentrated with mitochondria to the point where its appearance is affected (it appears brown), serotonin suppresses the primary energy generating activity of the adipocyte. It does this by suppressing glucose uptake and blocking fat thermogenesis, to presumably keep glucose free for core functional tissues (i.e. the brain) during stressful situations.
In muscle, serotonin acts directly on muscle cells to promote glycolysis, in addition to promoting cytokine production a key feature of the inflammation that accompanies all affective disorders.
In the rat brain, the uptake of phosphorus and oxygen is inhibited by serotonin. Downstream, this causes a downregulation of overall energy production in the brain, and an upregulation of glycolysis. The authors of one study in particular remarked that this is evidence that “serotonin impedes brain function by inhibition of energy producing reactions”. In humans, this result holds. Furthermore, increased serotonin and glycolysis has a direct relationship with depression as measured by the Hamilton Depression Scale:
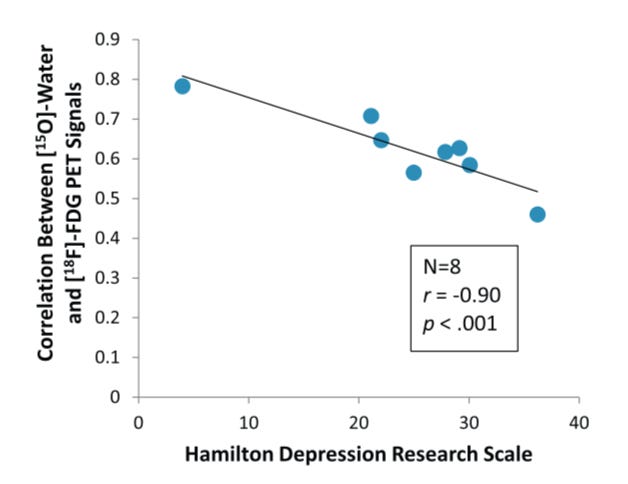
Figure credit: Drevets, 1992. A functional anatomical study of unipolar depression. J. Neurosci. 12, 3628–3641.
As the influx and conversion of oxygen to carbon dioxide in brain tissue goes down, signifying less phosphorus (ATP) production and increased glycolysis, patients report higher on the scale.
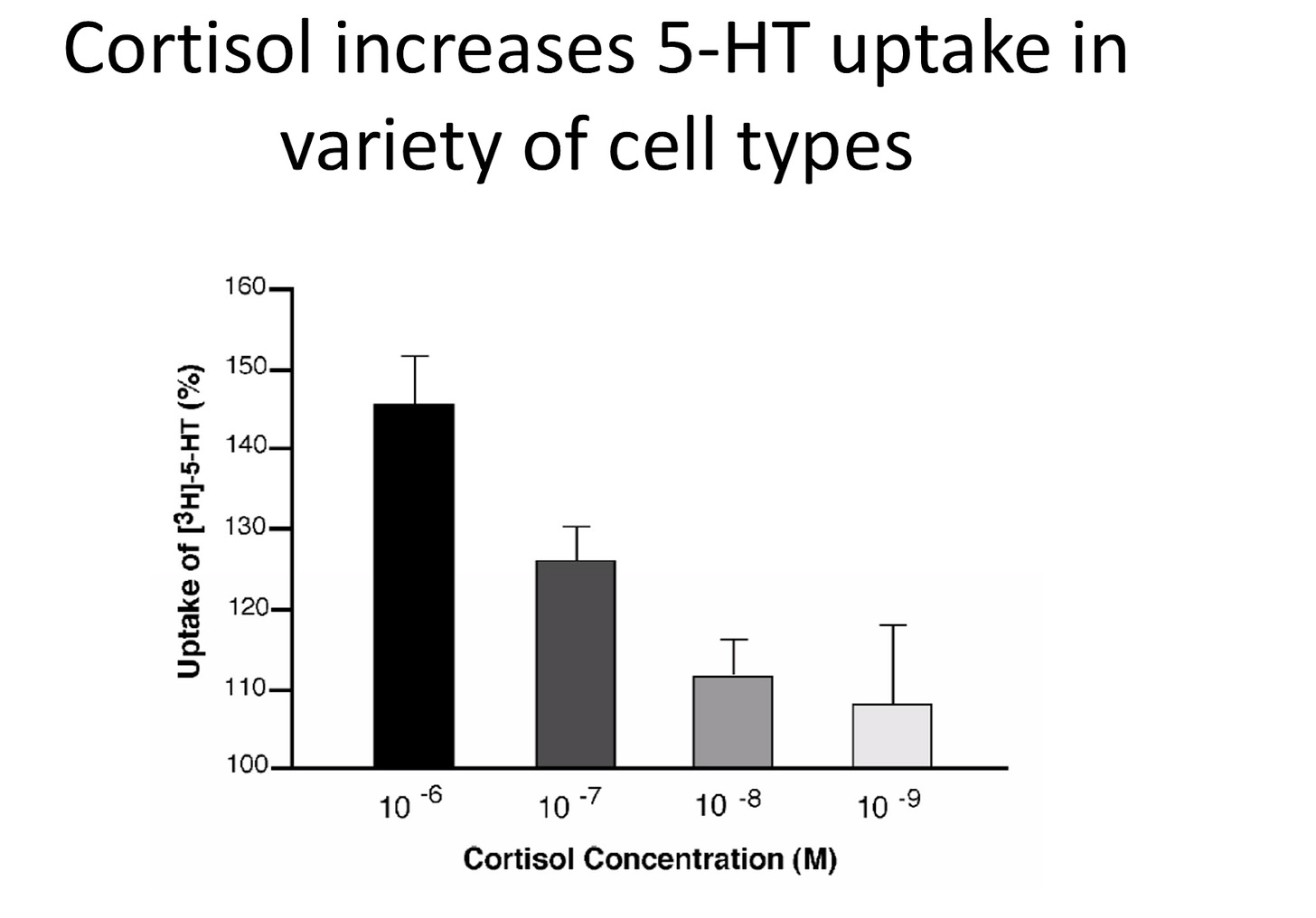
Figure credit: Tafet et al 2012
The piece that finally connects it all together is the evidence that cortisol increases serotonin uptake in a wide variety of cells, but particularly serotonin receptors that are found in the brain. As cortisol concentration increases, inflammation increases, and serotonin transmission increases. If you think back to the previous section where we (almost) definitively showed that increased cortisol is necessary and causative of depression, especially in experimental models, this should not be surprising at all, and fully expected.
There’s one last gap I think we can fill that I’ve only hinted at over the last few newsletters. If you’ve been keeping track, I keep emphasizing that serotonin seems to be less associated with feeling good (“the happy molecule”) and more often associated with stressful situations of high energy demand, or perceived stressful situations of high energy demand.
Rumination: How Thinking Real Hard and Often is a Costly But Adaptive Response to Stress, and A General Theory of Depression
Let’s link this altogether from end to end (or at least, I’ll attempt to). Given that:
people who suffer from mitochondrial disorders are also comorbid with depression at a fairly high rate (50-80% depending on the disease),
all clinically depressed people have disordered energy metabolism with respect to lactate production, inflammation, and glucose intolerance
Serotonin profoundly disrupts glucose metabolism by switching energy production in cells of almost all types it has been studied in to glycolysis from oxidative phosphorylation
And serotonin production increases in response to phenotypic states that correlate with lack of resources or perceived lack of resources (stress)
then, I would argue that serotonin’s role in the body is likely as a primary energy regulator in all tissues that it acts, specifically to help the organism adapt to stressful environmental demands. Furthermore, in humans, this role extends to all phenotypes we would qualitatively label as “depressed”, including major depressive disorder, starvation, sexual exhaustion, and rumination. In particular, it seems that rumination is an adaptation to complex situations which the brain cognitively parses as stressful, switching the energy production mode from oxidative phosphorylation to glycolysis, upregulating what we would call “depression”, in order to give you the ability to deal with the stress. This is accompanied by inflammation, mitochondrial degradation, and all the comorbidities that come along with inflammatory illnesses.
The body’s adaptive milieu confirms this position. The experimental way to induce depression is injection of cortisol, which causes systemic inflammation and raises serotonin, prolactin, estrogen, and other inflammatory stress hormones. Blocking cortisol directly via e.g. ketamine or indirectly via other hormones such as allopregnanolone has a demonstrated, proven, and rapid antidepressant effect.
It seems that the SSRIs that work all work as a result of increasing allopregnanolone by proxy, a phenomenon that no one has figured out mechanistically quite yet.
This is further bolstered by the observation that the therapeutic delay of all SSRIs is due to their acute insult on energy production caused by the increase of serotonin, which causes the body to adapt by producing more allopregnanolone, DHEA, and other hormones that assist in healthy oxidative metabolism.
So what causes depression? We’re left with a very complex picture to tug apart.
There’s decent evidence showing that energy is reallocated to cognition during the state of depression known as melancholia, at the expense of growth and reproduction, as we saw when we took a closer look at rumination. Melancholia is energetically expensive because it activates the HPA axis continuously, inhibiting sexual activity and growth. So why is melancholia, or clinical depression, doing this?
Rumination is, quoting Andrews et al, “persistent, distraction-resistant thoughts about the problems associated with [an episode of melancholia].” It is an analytical cognitive processing style where complex problems are broken down into smaller, manageable components, and sequentially studied. This analysis requires working memory in the brain, which is very costly. As the load on working memory increases, tasks can be interrupted more easily because things we’ve cognitively deemed irrelevant can displace task-specific information. It has been hypothesized that one of the ways we can power through this is by using rumination - it may promote analysis by reducing the vulnerability to interruption.
Rumination is an adaptation to complex, stressful situations. It has also been shown to increase glycolysis, which is in itself stressful, but is a compensatory response to stress. There are multiple ways to show that you’re switching energy production mechanisms, but among them are the core component of oxidative phosphorylation, cytochrome c oxidase activity, is majorly downregulated by stressed rodents, and the fact that in imaging studies of the brain, much of the product of glycolysis, lactate, escapes the reach of imaging. The hyperactivation of the HPA axis exhibited by all depressed animals demonstrates this further. It’s not that you are down when you’re depressed - it’s that you are hyper-stressed and ruminating constantly.
It is when this rumination intersects with a situation or situations from which you personally do not view a way out, that depression becomes major and clinical, in my opinion. How you are situated with respect to your circumstances, plays a major role in the etiology. The brain chemistry of “serotonin is a happy molecule” is merely a symptom, a lagging indicator. Everything else that cascades along with it, is also just a lagging indicator.
I’ll try to restate this in an easier, more straightforward way. When you perceive your situation as problematic and inescapable, your brain can react by upregulating ruminative behavior. This behavior is adaptive given the situation, and shifts the energy production of the brain from oxidative means to glycolytic means. This is accompanied by a surging in HPA axis activity, and accompanying stress hormones - cortisol, prolactin, estrogen, and most importantly, serotonin. Serotonin primarily is involved in assisting with this global shift in energy production, by, in some cases, literally turning on glycolysis. From this point of view rumination can be considered not only a symptom of depression but an attempt to adapt a heavy subjective challenge.
There are some wrinkles here, such as genotypes and mutations that may increase or decrease likelihood of serotonin transport, but a lot of that work has recently almost comprehensively been invalidated. There are others with respect to prenatal imprinting of estrogen production, serotonin production, cortisol production, etc, that I also won’t get into because these approaches are in their infancy and can’t explain much of what we observe in depressed people.
And there it is. I know it’s convoluted, and not at all simple, but if we accept the totality of the observed evidence, then it means that we’ve expanded greatly the realm of acceptable solutions. We can think far beyond influencing just serotonin as the happy molecule. We can consider environmental interventions. Societal interventions. Mental health can become a public policy issue again. Universal basic income can become a public mental health policy intervention.
Anti-cortisol drugs like ketamine, which are already approved for depression, can become formalized, science-based ways to address depression at scale.
The mitochondrial cocktail (riboflavin, vitamin E, L-carnitine, CoQ10, etc) can become a cheap, and widely scalable approach.
We will cover these, and many more possible interventions, in the last installment. Thanks for reading!
Thanks for subscribing! If you would like to share this newsletter with anyone, you can suggest they sign up here.
Journal of Neuroscience. “Peptide Inhibitors Disrupt the Serotonin 5-HT2C Receptor Interaction with Phosphatase and Tensin Homolog to Allosterically Modulate Cellular Signaling and Behavior”. Anastasio NC, Gilbertson SR, Bubar MJ, Agarkov A, Stutz SJ, Jeng Y, Bremer NM, Smith TD, Fox RG, Swinford SE, Seitz PK, Charendoff MN, Craft JW Jr, Laezza FM, Watson CS, Briggs JM, Cunningham KA.
Pharmacol Biochem Behav. 1993 Sep;46(1):9-13. “Involvement of brain tryptophan hydroxylase in the mechanism of hibernation.” Popova NK, Voronova IP, Kulikov AV.
Pharmacol Biochem Behav. 1981 Jun;14(6):773-7. “Brain serotonin metabolism in hibernation.” Popova NK, Voitenko NN.
J Comp Physiol B. “Mitochondrial metabolism in hibernation and daily torpor: a review.” Staples JF, Brown JC.
J Comp Physiol B. “Life in the fat lane: seasonal regulation of insulin sensitivity, food intake, and adipose biology in brown bears.” Rigano KS, Gehring JL, Evans Hutzenbiler BD, Chen AV, Nelson OL, Vella CA, Robbins CT, Jansen HT.
Science. “Serotonin mediates behavioral gregarization underlying swarm formation in desert locusts.” Anstey ML, Rogers SM, Ott SR, Burrows M, Simpson SJ.
Am J Physiol. “Does serotonin play a role in entrance into hibernation?” Canguilhem B, Miro JL, Kempf E, Schmitt P.
Genes, Brain, and Behavior. “The brain 5‐HT1A receptor gene expression in hibernation” V. S. Naumenko S. E. Tkachev A. V. Kulikov T. P. Semenova Z. G. Amerhanov N. P. Smirnova N. K. Popova.
Int. Conf. Bear Res. and Manage. “Insulin and Glucagon Responses in the Hibernating Black Bear.” PJ Palumbo.
Charney, D.S., Menkes, D.B., Heninger, G.R., 1981. Receptor sensitivity and the mech- anism of action of antidepressant treatment: implications for the etiology and therapy of depression. Arch. Gen. Psychiatry 38, 1160–1180.
Oswald, I., Brezinova, V., Dunleavy, D.L.F., 1972. On the slowness of action of tricyclic antidepressant drugs. Br. J. Psychiatry 120, 673–677.
Davies, D.L., Shepherd, M., 1955. Reserpine in the treatment of anxious and depressed patients. Lancet 266, 117–120.
Baumeister, A.A., Hawkins, M.F., Uzelac, S.M., 2003. The myth of reserpine-induced depression: role in the historical development of the monoamine hypothesis. J. Hist. Neurosci. 12, 207–220.
Kanes, Stephen J et al. “Open-label, proof-of-concept study of brexanolone in the treatment of severe postpartum depression.” Human psychopharmacology vol. 32,2 (2017): e2576. doi:10.1002/hup.2576
Martin P, Gozlan H, Puech AJ , Eur J Pharmacol 1992 Feb 25;212(1):73-8. 5-HT3 receptor antagonists reverse helpless behaviour in rats. Departement de Pharmacologie, Faculte de Medecine Pitie-Salpetriere, Paris, France.
Sustained rescue of prefrontal circuit dysfunction by antidepressant-induced spine formation. BY R. N. MODA-SAVA, M. H. MURDOCK, P. K. PAREKH, R. N. FETCHO, B. S. HUANG, T. N. HUYNH, J. WITZTUM, D. C. SHAVER, D. L. ROSENTHAL, E. J. ALWAY, K. LOPEZ, Y. MENG, L. NELLISSEN, L. GROSENICK, T. A. MILNER, K. DEISSEROTH, H. BITO, H. KASAI, C. LISTON. SCIENCE12 APR 2019
Bymaster, F.P., Zhang, W., Carter, P.A., Shaw, J., Chernet, E., Phebus, L., Wong, D.T., Perry, K.W., 2002. Fluoxetine, but not other selective serotonin uptake inhibitors, increases norepinephrine and dopamine extracellular levels in prefrontal cortex. Psychopharmacology (Berl) 160, 353–361.
Wang, Wei, et al. “Ketamine Improved Depressive-like Behaviors via Hippocampal Glucocorticoid Receptor in Chronic Stress Induced- Susceptible Mice.” Behavioural Brain Research, vol. 364, 2019, pp. 75–84., doi:10.1016/j.bbr.2019.01.057.
Vargiu, Lidia & Stefanini, E & Musinu, C & Saba, G. (1978). Possible role of brain serotonin in the central effects of ketamine. Neuropharmacology. 17. 405-8. 10.1016/0028-3908(78)90014-X.
Ruhe, H.G., Mason, N.S., Schene, A.S., 2007. Mood is indirectly related to serotonin,
norepinephrine and dopamine levels: a meta-analysis of monoamine depletion studies. Mol. Psychiatry 12, 331–359.
Andrews PW, Thomson Jr JA. The bright side of being blue: depression as an adaptation for analyzing complex problems. Psychol Rev 2009;116:620–54. Review.
Sumara,G., Sumara,O., Kim,J.K., and Karsenty,G.(2012)Gut-derivedserotonin is a multifunctional determinant to fasting adaptation. Cell Metab. 16, 588– 600.
Holmes, A., Murphy, D.L., Crawley, J.N., 2003. Abnormal behavioral phenotypes of serotonin transporter knockout mice: parallels with human anxiety and depression. Biol. Psychiatry 54, 953–959.
Coelho, W.S., Costa, K.C. and Sola-Penna, M. (2007) Serotonin stimulates mouse skeletal muscle 6-phosphofructo-1-kinase through tyrosine- phosphorylation of the enzyme altering its intracellular localization. Mol. Genet. Metab. 92, 364–370.
Coelho, W.S. and Sola-Penna, M. (2013) Serotonin regulates 6-phosphofructo- 1-kinase activity in a PLC-PKC-CaMK II- and Janus kinase-dependent signaling pathway. Mol. Cell. Biochem. 372, 211–220.
Fava M, et al. CLARITY: A phase 2 double-blind, placebo-controlled study to evaluate the efficacy and safety of adjunctive pimavanserin in major depressive disorder. Presented at: APA Annual Meeting; May 18-23, 2019; San Francisco.
Andrews, Paul & R Lee, Kyuwon & Fox, Molly & Bharwani, Aadil & Anderson Thomson Jr, J. (2015). Is serotonin an upper or a downer? The evolution of the serotonergic system and its role in depression and the antidepressant response. Neuroscience & Biobehavioral Reviews. 51. 164-188. 10.1016/j.neubiorev.2015.01.018.
Feenstra, M.G.P., van Galen, H., Te Riele, P.J.M., Botterblom, M.H.A., Mirmiran, M., 1996. Decreased hypothalamic serotonin levels in adult rats treated neonatally with clomipramine. Pharmacol. Biochem. Behav. 55, 647–652.
Hilakivi, L.A., Stenberg, D., Sinclair, J.D., Kiianmaa, K., 1987. Neonatal desipramine or zimeldine treatment causes long-lasting changes in brain monoaminergic systems and alcohol related behavior in rats. Psychopharmacology (Berl) 91, 403–409.
Ansorge, M.S., Zhou, M., Lira, A., Hen, R., Gingrich, J.A., 2004. Early-life blockade of the 5-HT transporter alters emotional behavior in adult mice. Science 306, 879–881.
Hansen, H., Sanchez, C., Meier, E., 1997. Neonatal administration of the selective serotonin reuptake inhibitor Lu 10-134-C increases forced swimming-induced immobility in adult rats: a putative animal model of depression? J. Pharmacol. Exp. Ther. 283, 1333–1341.
Zangen, A., Overstreet, D.H., Yadid, G., 1997. High serotonin and 5- hydroxyindoleacetic acid levels in limbic brain regions in a rat model of depression: normalization by chronic antidepressant treatment. J. Neurochem. 69, 2477–2483.
Iyer, L.M., Aravind, L., Coon, S.L., Klein, D.C., Koonin, E.V., 2004. Evolution of cell–cell signaling in animals: did late horizontal gene transfer from bacteria have a role? Trends Genet. 20, 292–299.
Gardner, Ann & G Boles, Richard. (2011). Beyond the serotonin hypothesis: Mitochondria, inflammation and neurodegeneration in major depression and affective spectrum disorders. Progress in neuro-psychopharmacology & biological psychiatry. 35. 730-43. 10.1016/j.pnpbp.2010.07.030.
McKenzie M, Liolitsa D, Hanna MG. Mitochondrial disease: mutations and mechanisms. Neurochem Res 2004;29(3):589–600.
Gardner A, Pagani M, Wibom R, Nennesmo I, Jacobsson H, Hällström T. Alterations of rCBF and mitochondrial dysfunction in major depressive disorder: a case report. Acta Psychiatr Scand 2003a;107(3):233–9.
Fattal O, Link J, Quinn K, Cohen BH, Franco K. Psychiatric comorbidity in 36 adults with mitochondrial cytopathies. CNS Spectr 2007;12(6):429–38.
Boles RG, Burnett BB, Gleditsch K, Wong S, Guedalia A, Kaariainen A, et al. A high predisposition to depression and anxiety in mothers and other matrilineal relatives of children with presumed maternally inherited mitochondrial disorders. Am J Med Genet B Neuropsychiatr Genet 2005;137B(1):20–4.
Burnett BB, Gardner A, Boles RG. Mitochondrial inheritance in depression, dysmotility and migraine? J Affect Disord 2005;88(1):109–16.
Sabunciyan S, Kirches E, Krause G, Bogerts B, Mawrin C, Llenos IC, et al. Quantification of total mitochondrial DNA and mitochondrial common deletion in the frontal cortex of patients with schizophrenia and bipolar disorder. J Neural Transm 2007;114(5): 665–74.
Shao L, Martin MV, Watson SJ, Schatzberg A, Akil H, Myers RM, et al. Mitochondrial involvement in psychiatric disorders. Ann Med 2008;40(4):281–95.
Stine OC, Luu SU, Zito M, Casanova M. The possible association between affective disorder and partially deleted mitochondrial DNA. Biol Psychiatry 1993;33(2): 141–2.
Gardner A, Johansson A, Wibom R, Nennesmo I, von Döbeln U, Hagenfeldt L, et al. Alterations of mitochondrial function and correlations with personality traits in selected major depressive disorder patients. J Affect Disord 2003b;76(1–3):55–68.
Gardner A, Boles RG. Mitochondrial energy depletion in depression with somatization. Psychother Psychosom 2008a;77(2):127–9.
Maes M, Yirmyia R, Noraberg J, Brene S, Hibbeln J, Perini G, et al. The inflammatory & neurodegenerative (I&ND) hypothesis of depression: leads for future research and new drug developments in depression. Metab Brain Dis 2009a;24(1):27–53.
Rodriguez MC, MacDonald JR, Mahoney DJ, Parise G, Beal MF, Tarnopolsky MA. Beneficial effects of creatine, CoQ10, and lipoic acid in mitochondrial disorders. Muscle Nerve 2007;35(2):235–42
Maes M, De Vos N, Pioli R, Demedts P, Wauters A, Neels H, et al. Lower serum vitamin E concentrations in major depression. Another marker of lowered antioxidant defenses in that illness. J Affect Disord 2000;58(3):241–6
Maes M, Yirmyia R, Noraberg J, Brene S, Hibbeln J, Perini G, et al. The inflammatory & neurodegenerative (I&ND) hypothesis of depression: leads for future research and new drug developments in depression. Metab Brain Dis 2009a;24(1):27–53
Pettegrew JW, Levine J, McClure RJ. Acetyl-L-carnitine physical-chemical, metabolic, and therapeutic properties: relevance for its mode of action in Alzheimer's disease and geriatric depression. Mol Psychiatry 2000;5(6):616–32.
Parikh S, Saneto R, Falk MJ, Anselm I, Cohen BH, Haas R. A modern approach to the treatment of mitochondrial disease. Curr Treat Options Neurol 2009;11(6):414–30.
Tarnopolsky MA. The mitochondrial cocktail: rationale for combined nutraceutical therapy in mitochondrial cytopathies. Adv Drug Deliv Rev 2008;60(13–14):1561–7.
Gold DR, Cohen BH. Treatment of mitochondrial cytopathies. Semin Neurol 2001;21(3): 309–25.
El merahbi, Rabih & Löffler, Mona & Mayer, Alexander & Sumara, Grzegorz. (2015). The roles of peripheral serotonin in metabolic homeostasis. FEBS letters. 589. 10.1016/j.febslet.2015.05.054.
J Mahler, D & L Humoller, F. (1968). The Influence of Serotonin on Oxidative Metabolism of Brain Mitochondria. Proceedings of the Society for Experimental Biology and Medicine. Society for Experimental Biology and Medicine (New York, N.Y.). 127. 1074-9. 10.3181/00379727-127-32874.
Drevets, W.C., Videen, T.O., Price, J.L., Preskorn, S.H., Carmichael, S.T., Raichle, M.E., 1992. A functional anatomical study of unipolar depression. J. Neurosci. 12, 3628–3641.
Kane, M.J., Engle, R.W., 2002. The role of prefrontal cortex in working-memory capacity, executive attention, and general fluid intelligence: an individual-differences perspective. Psychon. Bull. Rev. 9, 637–671.
Kanarik, M., Alttoa, A., Matrov, D., Kõiv, K., Sharp, T., Panksepp, J., Harro, J., 2011. Brain responses to chronic social defeat stress: effects on regional oxidative metabolism as a function of a hedonic trait, and gene expression in susceptible and resilient rats. Eur. Neuropsychopharmacol. 21, 92–107.
Shumake, J., Edward, E., Gonzalez-Lima, F., 2002. Dissociation of septo-hippocampal metabolism in the congenitally helpless rat. Neuroscience 114, 373–377.
Shumake, J., Edwards, E., Gonzalez-Lima, F., 2001. Hypermetabolism of paraventricular hypothalamus in the congenitally helpless rat. Neurosci. Lett. 311, 45–48.
Shumake, J., Edwards, E., Gonzalez-Lima, F., 2003. Opposite metabolic changes in the habenula and ventral tegmental area of a genetic model of helpless behavior. Brain Res. 963, 274–281.
Shumake, J., Poremba, A., Edwards, E., Gonzalez-Lima, F., 2000. Congenital helpless rats as a genetic model for cortex metabolism in depression. Neuroreport 11, 3793–3798.
Stone, E.A., Lehmann, M.L., Lin, Y., Quartermain, D., 2007. Reduced evoked fos expression in activity-related brain regions in animal models of behavioral depression. Prog. Neuropsychopharmacol. Biol. Psychiatry 31, 1196–1207.
Dienel, G.A., 2012. Brain lactate metabolism: the discoveries and the controversies. J. Cereb. Blood Flow Metab. 32, 1107–1138.
Tafet, Gustavo & P. Idoyaga-Vargas, Victor & P. Abulafia, Denise & Calandria, Jorgelina & S. Roffman, Silvia & Chiovetta, Amabella & Shinitzky, Meir. (2001). Correlation between cortisol level and serotonin uptake in patients with chronic stress and depression. Cognitive, affective & behavioral neuroscience. 1. 388-93. 10.3758/CABN.1.4.388.